Using low-bandgap III-V materials such as InAsSb in nanostructured arrays to limit potential loss mechanisms, a 25x improvement in mass specific power and ten thousands times decrease in volume from a MMRTG is an early estimate, with higher performance possible depending on operating conditions. TRC technology will allow a proliferation of small versatile spacecraft with power requirements not met by photovoltaic arrays or bulky, inefficient MMRTG systems. This will directly enable small-sat missions to the outer planets as well as operations in permanent shadow such as polar lunar craters.
They will build on the Phase I study which already showed the system works and generates power over 4 times better than traditional nuclear RTG power. They showed 8 W of electrical power is possible from the 62.5 W Pu-238 pellet from a general purpose heat source using a 0.28 eV bandgap TRC operating at 600 K. The necessary array includes 1,125 cm² of TRC emitters, or just over 50% of the surface area of a 6U cubesat. With a mass (heat source + TRC) of 622 g, a mass specific power of 12.7 W/kg is possible, over a 4.5x improvement from heritage multi-mission radioisotope thermoelectric generator (MMRTG) was shown.
This technology will allow a proliferation of small versatile spacecraft with power requirements not met by photovoltaic arrays or bulky, inefficient MMRTG systems. This will directly enable small-sat missions to the outer planets as well as operations in permanent shadow such as polar lunar craters. This study will investigate the thermodynamics and feasibility of the development of a radioisotope enabled thermoradiative power source focusing on system size, weight, power (SWaP) as well as materials growth of identified materials including InAsSb or InPSb by metalorganic vapor phase epitaxy. They will analyze a thermoradiative converter to power a cubesat (or fleet of cubesats) that can ride along with a Flagship Uranus mission, doing such tasks as serving as information relay for atmospheric probes, and getting a parallax view of the planet and moons
This study will investigate the thermodynamics and feasibility of the development of a radioisotope enabled thermoradiative power source focusing on system size, weight, power (SWaP) while continuing to integrate the effects of potential power and efficiency loss mechanisms developed in Phase I. Experimentally, materials and TRC devices will be grown including InAsSb-based type-II superlattices by metalorganic vapor phase epitaxy (MOVPE) to target low-bandgap materials with suppressed Auger recombination.
Metal-semiconductor contacts capable of surviving the required elevated temperatures will be investigated. TRC devices will be tested for performance at elevated temperature facing a cold ambient under vacuum in a modified cryostat testing apparatus developed in Phase I.
We will analyze a radioisotope thermoradiative converter to power a cubesat mission operating at Uranus. This will include an engineering design study of our reference mission with the Compass engineering team at NASA Glenn Research Center with expertise on the impact of new technologies on spacecraft design in the context of an overall mission, incorporating all engineering disciplines and combining them at a system level. Finally, we will develop a technological roadmap for the necessary components of the TRC to power a future mission.
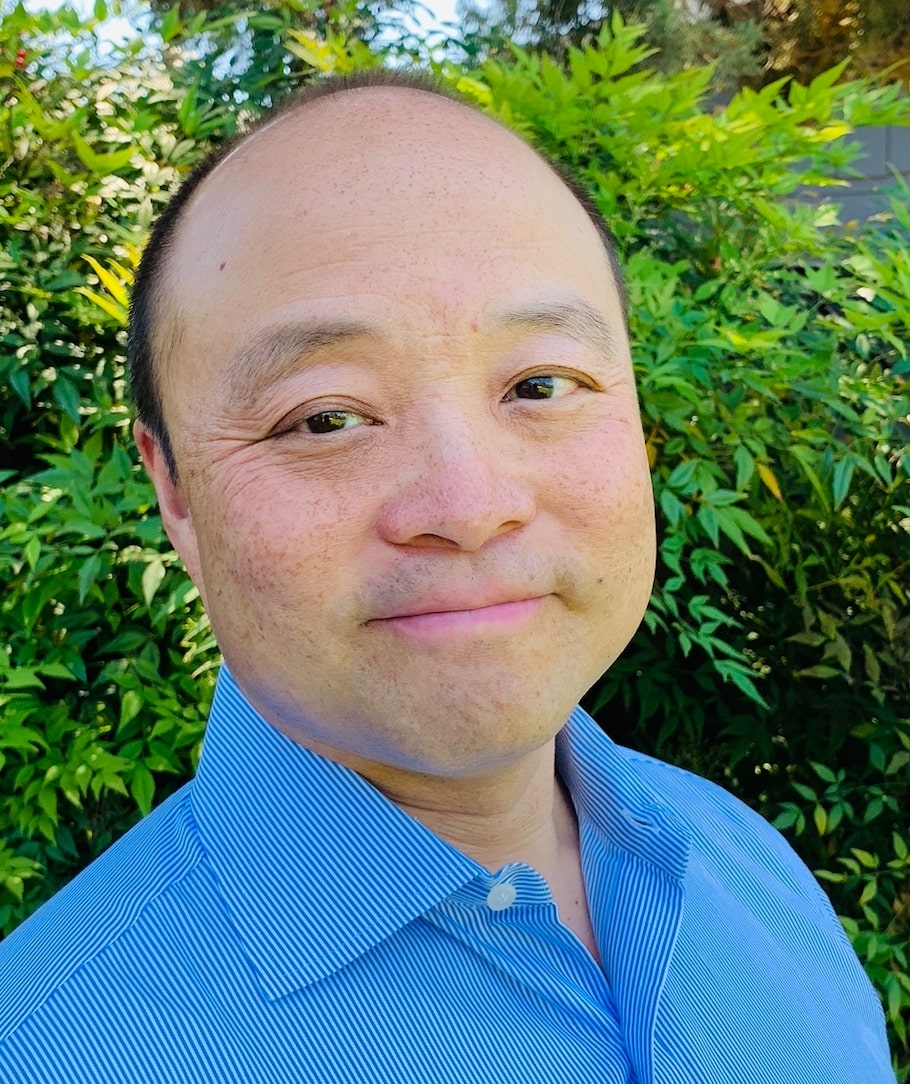
Brian Wang is a Futurist Thought Leader and a popular Science blogger with 1 million readers per month. His blog Nextbigfuture.com is ranked #1 Science News Blog. It covers many disruptive technology and trends including Space, Robotics, Artificial Intelligence, Medicine, Anti-aging Biotechnology, and Nanotechnology.
Known for identifying cutting edge technologies, he is currently a Co-Founder of a startup and fundraiser for high potential early-stage companies. He is the Head of Research for Allocations for deep technology investments and an Angel Investor at Space Angels.
A frequent speaker at corporations, he has been a TEDx speaker, a Singularity University speaker and guest at numerous interviews for radio and podcasts. He is open to public speaking and advising engagements.
@Scaryjello – this is neither thermoelectric or thermophotovoltaic, but “thermoradiative”, which I had not previously encountered. There is a conference paper directly related to this article but it’s behind an IEEE paywall. A 2022 paper by NASA’s Geoffrey Landis outlines the theoretical basis and it’s free on Researchgate. “Thermoradiative Arrays: A New Technology for Conversion of Heat into Electrical Power”. It’s an interesting read. I’m guessing the recent press release relates to some lab verification work of the theory, but can’t find details. I’m guessing a very small-scale demonstration of concept has been achieved, but no more.
Pu238 is expensive to produce. It’s a wonderful source of power. We need to explore more cost-effective means of production. The other thing we need to develop is small space reactors. Once developed and mass produced they could be much cheaper.
The question is long term durability. I mean semiconductors tend to degrade, especially if the temperature is high. Everybody knows that who has built A class power amp. There are a lot of factor what result it, roughly the same appear in photovoltaic cells.
Very limited production / availability of Pu 238 — the optimal energy source for RTG — may put a crimp in the number of long duration missions that can be deployed. I’ve long forgotten the sequence of nuclear reactions and decays needed to produce it, but I know that the small supply we have is a byproduct of nuclear weapons production. There are other radioisotopes that are much easier to produce, but they tend to have short half-lives that make them useless for long duration missions. The few that don’t have short half-lives tend to have very long half-lives, which means that a large mass of the isotope is needed to generate a useful amount of power. Pu 238 is the only isotope with a half-life in the Goldilocks zone suited for long duration missions. 87.7 years, according to Google. The next best choice, I believe, has a half-life of about 12 years — IIRC. But I’ve forgotten what the element is.
I think there is a fission product beta emitter like Sr90 that has been used in RTG in the USSR; somebody from Vanderbilt was interviewed on Atomic Insights trying to commercialize that tech; I recall there was an admiral on the advisory board. Honestly, we could use kg/kilowatt scale fission (UO2 in FeCrAl) as a substitute gram/watt scale 238Pu alpha decay, if the use case was there (i.e. NEED instead of WANT).
This is thermophotoelectric conversion tech that has been invented, made, published, demonstrated and working for at least 20 years now, with efficiencies in the 15~25% range minimum, depending on the optimisations applied. State of the art is 40%+ and published in Nature in 2022: 10.1038/s41586-022-04473-y
Someone in NASA finally got clearance to access google and wikipedia, but not yet the funding for Nature subscription. 13% is not so bad for the first try, but really it would be better if someone got Mr Musk interested in this. May come handy on Mars too.
Your Nature article describes a “thermophotovoltaic”, being a low-eV photovoltaic, but still a photovoltaic needing 1900-2400 deg-C emitter (light source).
RTG use thermoelectric (seebeck/peltier) devices and a temperature gradient, not light.
So, if NASA has a 13% efficient thermoelectric at 600 kelvin, it’s a big deal because even a PWR can make 600 kelvin (620 F).
So long as there is no gimmick or unobtanium involved it could find a lot of uses. The lead-telluride used previously just a dumb crystal that made with ground up reactants grown in a quartz tube under resistance heating.
Let me simplify it for you. There is nothing “thermoelectric” or “Seeback/Peltier” or “lead-telluride” or “silicon-germanium” (NASA favourite) here – neither in this new NASA work, nor in the earlier work by people who created this field and reached 40% conversion efficiency 2 years ago. The only difference with heterojunction solar cells is use of infrared spectrum only, and the materials to make this conversion. New for NASA, old for everyone else interested in this field.
Yes, it can find a lot of uses, especially with selective reflectors that pass only the useful photons (narrow band within infrared spectrum with highest conversion efficiency). Simply burning anything hot enough to make 3000C radiator glow at 40% efficient converter makes an awful lot of things obsolete. This is also not new, none of this is, except for NASA.
Shoot getting 12% efficiency out of a peltier (as opposed to 3% for lead telluride) makes an attractive top cycle for terrestrial use also. There’s got to be a catch
8W/62W = 13% efficiency is fantastic. 10% would be wonderful. Imagine what could be done with 100kWe in space… just using ion propulsion